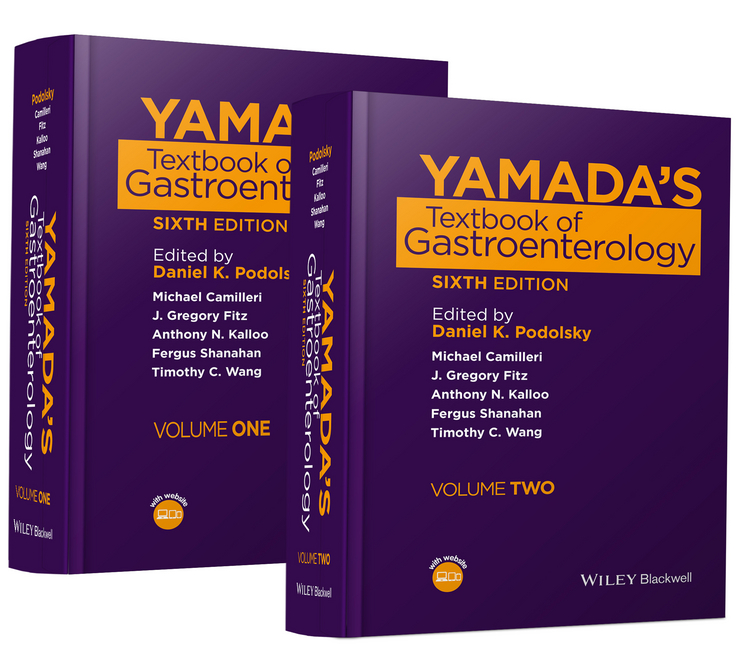
Edited by Daniel K. Podolsky, Michael Camilleri, J.
Gregory Fitz,
Anthony
N. Kalloo, Fergus Shanahan, Timothy C. Wang
1. RobytJF, FrenchD. The action pattern of porcine pancreatic alpha‐amylase in relationship to the substrate binding site of the enzyme. J Biol Chem1970;245:3917.
2. GrayGM. Starch digestion and absorption in nonruminants. J Nutr1992;122:172.
3. Van BeersEH, BullerHA, GrandRJ, et al.Intestinal brush border glycohydrolases: structure, function, and development. Crit Rev Biochem Mol Biol1995;30:197. CrossRef
4. Gudmand‐HoyerE, SkovbjergH. Disaccharide digestion and maldigestion. Scand J Gastroenterol Suppl1996;216:111. CrossRef
5. NajjarSM, HamppLT, RabkinR, et al.Sucrase‐alpha‐dextrinase in diabetic BioBreed rats: reversible alteration of subunit structure. Am J Physiol1991;260:G275.
6. NaimHY, RothJ, SterchiEE, et al.Sucrase‐isomaltase deficiency in humans. Different mutations disrupt intracellular transport, processing, and function of an intestinal brush border enzyme. J Clin Invest1988;82:667. CrossRef
7. TraberPG. Regulation of sucrase‐isomaltase gene expression along the crypt‐villus axis of rat small intestine. Biochem Biophys Res Commun1990;173:765. CrossRef
8. TreemWR. Clinical aspects and treatment of congenital sucrase‐isomaltase deficiency. J Pediatr Gastroenterol Nutr2012;55(Suppl. 2):S7. CrossRef
9. TreemWR. Clinical heterogeneity in congenital sucrase‐isomaltase deficiency. J Pediatr1996;128:727. CrossRef
10. TreemWR, McAdamsL, StanfordL, et al.Sacrosidase therapy for congenital sucrase‐isomaltase deficiency. J Pediatr Gastroenterol Nutr1999;28:137. CrossRef
11. BullerHA, KotheMJ, GoldmanDA, et al.Coordinate expression of lactase‐phlorizin hydrolase mRNA and enzyme levels in rat intestine during development. J Biol Chem1990;265:6978.
12. NaimHY, SterchiEE, LentzeMJ. Biosynthesis and maturation of lactase‐phlorizin hydrolase in the human small intestinal epithelial cells. Biochem J1987;241:427. CrossRef
13. RingsEH, KrasinskiSD, van BeersEH, et al.Restriction of lactase gene expression along the proximal‐to‐distal axis of rat small intestine occurs during postnatal development. Gastroenterology1994;106:1223.
14. BullerHA, GrandRJ. Lactose intolerance. Annu Rev Med1990;41:141. CrossRef
15. KuokkanenM, KokkonenJ, EnattahNS, et al.Mutations in the translated region of the lactase gene (LCT) underlie congenital lactase deficiency. Am J Hum Genet2006;78:339. CrossRef
16. WangY, HarveyCB, HolloxEJ, et al.The genetically programmed down‐regulation of lactase in children. Gastroenterology1998;114:1230. CrossRef
17. FlatzG. Genetics of lactose digestion in humans. Adv Hum Genet1987;16:1. CrossRef
18. EnattahNS, SahiT, SavilahtiE, et al.Identification of a variant associated with adult‐type hypolactasia. Nat Genet2002;30:233. CrossRef
19. KellyJJ, AlpersDH. Properties of human intestinal glucoamylase. Biochim Biophys Acta1973;315:113. CrossRef
20. NaimHY, SterchiEE, LentzeMJ. Structure, biosynthesis, and glycosylation of human small intestinal maltase‐glucoamylase. J Biol Chem1988;263:19709.
21. LebenthalE, Khin MaungU, ZhengBY, et al.Small intestinal glucoamylase deficiency and starch malabsorption: a newly recognized alpha‐glucosidase deficiency in children. J Pediatr1994;124:541. CrossRef
22. GalandG. Brush border membrane sucrase‐isomaltase, maltase‐glucoamylase and trehalase in mammals. Comparative development, effects of glucocorticoids, molecular mechanisms, and phylogenetic implications. Comp Biochem Physiol B1989;94:1. CrossRef
23. GartnerH, ShuklaP, MarkesichDC, et al.Developmental expression of trehalase: role of transcriptional activation. Biochim Biophys Acta2002;1574:329. CrossRef
24. Gudmand‐HoyerE, FengerHJ, SkovbjergH, et al.Trehalase deficiency in Greenland. Scand J Gastroenterol1988;23:775. CrossRef
25. LuD, YuanX, ZhengX, et al.Bovine proenteropeptidase is activated by trypsin, and the specificity of enteropeptidase depends on the heavy chain. J Biol Chem1997;272:31293. CrossRef
26. TownesPL. Trypsinogen deficiency disease. J Pediatr1965;66:275. CrossRef
27. HolzingerA, MaierEM, BuckC, et al.Mutations in the proenteropeptidase gene are the molecular cause of congenital enteropeptidase deficiency. Am J Hum Genet2002;70:20. CrossRef
28. YoshiokaM, EricksonRH, WoodleyJF, et al.Role of rat intestinal brush‐border membrane angiotensin‐converting enzyme in dietary protein digestion. Am J Physiol1987;253:G781.
29. MoritaA, ChungYC, FreemanHJ, et al.Intestinal assimilation of a proline‐containing tetrapeptide. Role of a brush border membrane postproline dipeptidyl aminopeptidase IV. J Clin Invest1983;72:610. CrossRef
30. LupiA, TenniR, RossiA, et al.Human prolidase and prolidase deficiency: an overview on the characterization of the enzyme involved in proline recycling and on the effects of its mutations. Amino Acids2008;35:739. CrossRef
31. LiaoTH, HamoshP, HamoshM. Fat digestion by lingual lipase: mechanism of lipolysis in the stomach and upper small intestine. Pediatr Res1984;18:402. CrossRef
32. FinkCS, HamoshP, HamoshM. Fat digestion in the stomach: stability of lingual lipase in the gastric environment. Pediatr Res1984;18:248. CrossRef
33. AbramsCK, HamoshM, LeeTC, et al.Gastric lipase: localization in the human stomach. Gastroenterology1988;95:1460.
34. AbramsCK, HamoshM, HubbardVS, et al.Lingual lipase in cystic fibrosis. Quantitation of enzyme activity in the upper small intestine of patients with exocrine pancreatic insufficiency. J Clin Invest1984;73:374. CrossRef
35. HamoshM, ScanlonJW, GanotD, et al.Fat digestion in the newborn. Characterization of lipase in gastric aspirates of premature and term infants. J Clin Invest1981;67:838. CrossRef
36. ArmandM, HamoshM, PhilpottJR, et al.Gastric function in children with cystic fibrosis: effect of diet on gastric lipase levels and fat digestion. Pediatr Res2004;55:457. CrossRef
37. CareyMC, SmallDM, BlissCM. Lipid digestion and absorption. Annu Rev Physiol1983;45:651. CrossRef
38. van TilbeurghH, BezzineS, CambillauC, et al.Colipase: structure and interaction with pancreatic lipase. Biochim Biophys Acta1999;1441:173. CrossRef
39. MattsonFH, VolpenheinRA. The digestion and absorption of triglycerides. J Biol Chem1964;239:2772.
40. LambeauG, GelbMH. Biochemistry and physiology of mammalian secreted phospholipases A2. Annu Rev Biochem2008;77:495. CrossRef
41. ArnesjoB, GrubbA. The activation, purification and properties of rat pancreatic juice phospholipase A 2. Acta Chem Scand1971;25:577. CrossRef
42. DiPersioLP, FontaineRN, HuiDY. Identification of the active site serine in pancreatic cholesterol esterase by chemical modification and site‐specific mutagenesis. J Biol Chem1990;265:16801.
43. DiPersioLP, FontaineRN, HuiDY. Site‐specific mutagenesis of an essential histidine residue in pancreatic cholesterol esterase. J Biol Chem1991;266:4033.
44. DiPersioLP, HuiDY. Aspartic acid 320 is required for optimal activity of rat pancreatic cholesterol esterase. J Biol Chem1993;268:300.
45. WrightEM. The intestinal Na+/glucose cotransporter. Annu Rev Physiol1993;55:575. CrossRef
46. TurkE, KernerCJ, LostaoMP, et al.Membrane topology of the human Na+/glucose cotransporter SGLT1. J Biol Chem1996;271:1925. CrossRef
47. WrightEM, LooDD. Coupling between Na+, sugar, and water transport across the intestine. Ann N Y Acad Sci2000;915:54. CrossRef
48. TurkE, ZabelB, MundlosS, et al.Glucose/galactose malabsorption caused by a defect in the Na+/glucose cotransporter. Nature1991;350:354. CrossRef
49. MartinMG, TurkE, LostaoMP, et al.Defects in Na+/glucose cotransporter (SGLT1) trafficking and function cause glucose‐galactose malabsorption. Nat Genet1996;12:216. CrossRef
50. ElsasLJ, LongoN. Glucose transporters. Annu Rev Med1992;43:377. CrossRef
51. KellettGL. The facilitated component of intestinal glucose absorption. J Physiol2001;531:585. CrossRef
52. DavidsonNO, HausmanAM, IfkovitsCA, et al.Human intestinal glucose transporter expression and localization of GLUT5. Am J Physiol1992;262:C795.
53. GouyonF, OnestoC, DaletV, et al.Fructose modulates GLUT5 mRNA stability in differentiated Caco‐2 cells: role of cAMP‐signalling pathway and PABP (polyadenylated‐binding protein)‐interacting protein (Paip) 2. Biochem J2003;375:167. CrossRef
54. WrightEM, MartinMG, TurkE. Intestinal absorption in health and disease – sugars. Best Pract Res Clin Gastroenterol2003;17:943. CrossRef
55. DuroD, RisingR, CedilloM, et al.Association between infantile colic and carbohydrate malabsorption from fruit juices in infancy. Pediatrics2002;109:797. CrossRef
56. ZieglerTR, Fernandez‐EstivarizC, GuLH, et al.Distribution of the H+/peptide transporter PepT1 in human intestine: up‐regulated expression in the colonic mucosa of patients with short‐bowel syndrome. Am J Clin Nutr2002;75:922.
57. TeradaT, InuiK. Peptide transporters: structure, function, regulation and application for drug delivery. Curr Drug Metab2004;5:85. CrossRef
58. GanapathyV, LeibachFH. Peptide transporters. Curr Opin Nephrol Hypertens1996;5:395. CrossRef
59. WuenschT, SchulzS, UllrichS, et al.The peptide transporter PEPT1 is expressed in distal colon in rodents and humans and contributes to water absorption. Am J Physiol Gastrointest Liver Physiol2013;305:G66. CrossRef
60. KletaR, RomeoE, RisticZ, et al.Mutations in SLC6A19, encoding B0AT1, cause Hartnup disorder. Nat Genet2004;36:999. CrossRef
61. RomeoE, DaveMH, BacicD, et al.Luminal kidney and intestine SLC6 amino acid transporters of B0AT‐cluster and their tissue distribution in Mus musculus. Am J Physiol Renal Physiol2006;290:F376. CrossRef
62. NozakiJ, DakeishiM, OhuraT, et al.Homozygosity mapping to chromosome 5p15 of a gene responsible for Hartnup disorder. Biochem Biophys Res Commun2001;284:255. CrossRef
63. SloanJL, MagerS. Cloning and functional expression of a human Na(+) and Cl(−)‐dependent neutral and cationic amino acid transporter B(0+). J Biol Chem1999;274:23740. CrossRef
64. KekudaR, Torres‐ZamoranoV, FeiYJ, et al.Molecular and functional characterization of intestinal Na(+)‐dependent neutral amino acid transporter B0. Am J Physiol1997;272:G1463.
65. KanaiY, HedigerMA. The glutamate/neutral amino acid transporter family SLC1: molecular, physiological and pharmacological aspects. Pflugers Arch2004;447:469. CrossRef
66. BaileyCG, RyanRM, ThoengAD, et al.Loss‐of‐function mutations in the glutamate transporter SLC1A1 cause human dicarboxylic aminoaciduria. J Clin Invest2011;121:446. CrossRef
67. Dello StrologoL, PrasE, PontesilliC, et al.Comparison between SLC3A1 and SLC7A9 cystinuria patients and carriers: a need for a new classification. J Am Soc Nephrol2002;13:2547. CrossRef
68. BarbosaM, LopesA, MotaC, et al.Clinical, biochemical and molecular characterization of cystinuria in a cohort of 12 patients. Clin Genet2012;81:47. CrossRef
69. PalacinM, NunesV, Font‐LlitjosM, et al.The genetics of heteromeric amino acid transporters. Physiology (Bethesda)2005;20:112. CrossRef
70. BorsaniG, BassiMT, SperandeoMP, et al.SLC7A7, encoding a putative permease‐related protein, is mutated in patients with lysinuric protein intolerance. Nat Genet1999;21:297. CrossRef
71. PinedaM, FernandezE, TorrentsD, et al.Identification of a membrane protein, LAT‐2, that Co‐expresses with 4F2 heavy chain, an L‐type amino acid transport activity with broad specificity for small and large zwitterionic amino acids. J Biol Chem1999;274:19738. CrossRef
72. TorrentsD, MykkanenJ, PinedaM, et al.Identification of SLC7A7, encoding y+LAT‐1, as the lysinuric protein intolerance gene. Nat Genet1999;21:293. CrossRef
73. ThamotharanM, BawaniSZ, ZhouX, et al.Hormonal regulation of oligopeptide transporter pept‐1 in a human intestinal cell line. Am J Physiol1999;276:C821.
74. AshidaK, KatsuraT, MotohashiH, et al.Thyroid hormone regulates the activity and expression of the peptide transporter PEPT1 in Caco‐2 cells. Am J Physiol Gastrointest Liver Physiol2002;282:G617. CrossRef
75. BrandschM, MiyamotoY, GanapathyV, et al.Expression and protein kinase C‐dependent regulation of peptide/H+ co‐transport system in the Caco‐2 human colon carcinoma cell line. Biochem J1994;299:253. CrossRef
76. EricksonRH, GumJRJr, LindstromMM, et al.Regional expression and dietary regulation of rat small intestinal peptide and amino acid transporter mRNAs. Biochem Biophys Res Commun1995;216:249. CrossRef
77. MerlinD, Si‐TaharM, SitaramanSV, et al.Colonic epithelial hPepT1 expression occurs in inflammatory bowel disease: transport of bacterial peptides influences expression of MHC class 1 molecules. Gastroenterology2001;120:1666. CrossRef
78. KeatingN, KeelySJ. Bile acids in regulation of intestinal physiology. Curr Gastroenterol Rep2009;11:375. CrossRef
79. WangTY, LiuM, PortincasaP, et al.New insights into the molecular mechanism of intestinal fatty acid absorption. Eur J Clin Invest2013;43:1203.
80. IqbalJ, HussainMM. Intestinal lipid absorption. Am J Physiol Endocrinol Metab2009;296:E1183. CrossRef
81. GimenoRE. Fatty acid transport proteins. Curr Opin Lipidol2007;18:271. CrossRef
82. LundgrenS, CarlingT, HjalmG, et al.Tissue distribution of human gp330/megalin, a putative Ca(2+)‐sensing protein. J Histochem Cytochem1997;45:383. CrossRef
83. WillnowTE, HilpertJ, ArmstrongSA, et al.Defective forebrain development in mice lacking gp330/megalin. Proc Natl Acad Sci U S A1996;93:8460. CrossRef
84. HoebeK, GeorgelP, RutschmannS, et al.CD36 is a sensor of diacylglycerides. Nature2005;433:523. CrossRef
85. PepinoMY, KudaO, SamovskiD, et al.Structure‐function of CD36 and importance of fatty acid signal transduction in fat metabolism. Annu Rev Nutr2014;34:281. CrossRef
86. LoboMV, HuertaL, Ruiz‐VelascoN, et al.Localization of the lipid receptors CD36 and CLA‐1/SR‐BI in the human gastrointestinal tract: towards the identification of receptors mediating the intestinal absorption of dietary lipids. J Histochem Cytochem2001;49:1253. CrossRef
87. Love‐GregoryL, ShervaR, SunL, et al.Variants in the CD36 gene associate with the metabolic syndrome and high‐density lipoprotein cholesterol. Hum Mol Genet2008;17:1695. CrossRef
88. AbumradNA, DavidsonNO. Role of the gut in lipid homeostasis. Physiol Rev2012;92:1061. CrossRef
89. HallAM, WiczerBM, HerrmannT, et al.Enzymatic properties of purified murine fatty acid transport protein 4 and analysis of acyl‐CoA synthetase activities in tissues from FATP4 null mice. J Biol Chem2005;280:11948. CrossRef
90. BergeKE, TianH, GrafGA, et al.Accumulation of dietary cholesterol in sitosterolemia caused by mutations in adjacent ABC transporters. Science2000;290:1771. CrossRef
91. DavisHRJr, ZhuLJ, HoosLM, et al.Niemann‐Pick C1 Like 1 (NPC1L1) is the intestinal phytosterol and cholesterol transporter and a key modulator of whole‐body cholesterol homeostasis. J Biol Chem2004;279:33586. CrossRef
92. AltmannSW, DavisHRJr, ZhuLJ, et al.Niemann‐Pick C1 Like 1 protein is critical for intestinal cholesterol absorption. Science2004;303:1201. CrossRef
93. HardieDG, RossFA, HawleySA. AMPK: a nutrient and energy sensor that maintains energy homeostasis. Nat Rev Mol Cell Biol2012;13:251. CrossRef
94. XiaoB, SandersMJ, UnderwoodE, et al.Structure of mammalian AMPK and its regulation by ADP. Nature2011;472:230. CrossRef
95. ScottJW, HawleySA, GreenKA, et al.CBS domains form energy‐sensing modules whose binding of adenosine ligands is disrupted by disease mutations. J Clin Invest2004;113:274. CrossRef
96. GowansGJ, HardieDG. AMPK: a cellular energy sensor primarily regulated by AMP. Biochem Soc Trans2014;42:71. CrossRef
97. HawleySA, PanDA, MustardKJ, et al.Calmodulin‐dependent protein kinase kinase‐beta is an alternative upstream kinase for AMP‐activated protein kinase. Cell Metab2005;2:9. CrossRef
98. BoudeauJ, ScottJW, RestaN, et al.Analysis of the LKB1‐STRAD‐MO25 complex. J Cell Sci2004;117:6365. CrossRef
99. DaviesSP, HelpsNR, CohenPT, et al.5′‐AMP inhibits dephosphorylation, as well as promoting phosphorylation, of the AMP‐activated protein kinase. Studies using bacterially expressed human protein phosphatase‐2C alpha and native bovine protein phosphatase‐2AC. FEBS Lett1995;377:421. CrossRef
100. HawleySA, RossFA, ChevtzoffC, et al.Use of cells expressing gamma subunit variants to identify diverse mechanisms of AMPK activation. Cell Metab2010;11:554. CrossRef
101. SanliT, RashidA, LiuC, et al.Ionizing radiation activates AMP‐activated kinase (AMPK): a target for radiosensitization of human cancer cells. Int J Radiat Oncol Biol Phys2010;78:221. CrossRef
102. DaleS, WilsonWA, EdelmanAM, et al.Similar substrate recognition motifs for mammalian AMP‐activated protein kinase, higher plant HMG‐CoA reductase kinase‐A, yeast SNF1, and mammalian calmodulin‐dependent protein kinase I. FEBS Lett1995;361:191. CrossRef
103. MarsinAS, BertrandL, RiderMH, et al.Phosphorylation and activation of heart PFK‐2 by AMPK has a role in the stimulation of glycolysis during ischaemia. Curr Biol2000;10:1247. CrossRef
104. HabetsDD, CoumansWA, El HasnaouiM, et al.Crucial role for LKB1 to AMPKalpha2 axis in the regulation of CD36‐mediated long‐chain fatty acid uptake into cardiomyocytes. Biochim Biophys Acta2009;1791:212. CrossRef
105. PehmollerC, TreebakJT, BirkJB, et al.Genetic disruption of AMPK signaling abolishes both contraction‐ and insulin‐stimulated TBC1D1 phosphorylation and 14‐3‐3 binding in mouse skeletal muscle. Am J Physiol Endocrinol Metab2009;297:E665. CrossRef
106. McCrimmonRJ, ShawM, FanX, et al.Key role for AMP‐activated protein kinase in the ventromedial hypothalamus in regulating counterregulatory hormone responses to acute hypoglycemia. Diabetes2008;57:444. CrossRef
107. JacksonRJ, HellenCU, PestovaTV. The mechanism of eukaryotic translation initiation and principles of its regulation. Nat Rev Mol Cell Biol2010;11:113. CrossRef
108. KilbergMS, BalasubramanianM, FuL, et al.The transcription factor network associated with the amino acid response in mammalian cells. Adv Nutr2012;3:295. CrossRef
109. BerlangaJJ, SantoyoJ, De HaroC. Characterization of a mammalian homolog of the GCN2 eukaryotic initiation factor 2alpha kinase. Eur J Biochem1999;265:754. CrossRef
110. KimballSR, JeffersonLS. Role of amino acids in the translational control of protein synthesis in mammals. Semin Cell Dev Biol2005;16:21. CrossRef
111. BrushMH, WeiserDC, ShenolikarS. Growth arrest and DNA damage‐inducible protein GADD34 targets protein phosphatase 1 alpha to the endoplasmic reticulum and promotes dephosphorylation of the alpha subunit of eukaryotic translation initiation factor 2. Mol Cell Biol2003;23:1292. CrossRef
112. VattemKM, WekRC. Reinitiation involving upstream ORFs regulates ATF4 mRNA translation in mammalian cells. Proc Natl Acad Sci U S A2004;101:11269. CrossRef
113. SiuF, BainPJ, LeBlanc‐ChaffinR, et al.ATF4 is a mediator of the nutrient‐sensing response pathway that activates the human asparagine synthetase gene. J Biol Chem2002;277:24120. CrossRef
114. ShanJ, OrdD, OrdT, et al.Elevated ATF4 expression, in the absence of other signals, is sufficient for transcriptional induction via CCAAT enhancer‐binding protein‐activating transcription factor response elements. J Biol Chem2009;284:21241. CrossRef
115. FawcettTW, MartindaleJL, GuytonKZ, et al.Complexes containing activating transcription factor (ATF)/cAMP‐responsive‐element‐binding protein (CREB) interact with the CCAAT/enhancer‐binding protein (C/EBP)‐ATF composite site to regulate Gadd153 expression during the stress response. Biochem J1999;339:135.
116. ThiavilleMM, DudenhausenEE, ZhongC, et al.Deprivation of protein or amino acid induces C/EBPbeta synthesis and binding to amino acid response elements, but its action is not an absolute requirement for enhanced transcription. Biochem J2008;410:473. CrossRef
117. ChantranupongL, WolfsonRL, SabatiniDM. Nutrient‐sensing mechanisms across evolution. Cell2015;161:67. CrossRef
118. LaplanteM, SabatiniDM. mTOR signaling in growth control and disease. Cell2012;149:274. CrossRef
119. KimDH, SarbassovDD, AliSM, et al.mTOR interacts with raptor to form a nutrient‐sensitive complex that signals to the cell growth machinery. Cell2002;110:163. CrossRef
120. WangL, HarrisTE, RothRA, et al.PRAS40 regulates mTORC1 kinase activity by functioning as a direct inhibitor of substrate binding. J Biol Chem2007;282:20036. CrossRef
121. JacintoE, LoewithR, SchmidtA, et al.Mammalian TOR complex 2 controls the actin cytoskeleton and is rapamycin insensitive. Nat Cell Biol2004;6:1122. CrossRef
122. SekiguchiT, HiroseE, NakashimaN, et al.Novel G proteins, Rag C and Rag D, interact with GTP‐binding proteins, Rag A and Rag B. J Biol Chem2001;276:7246. CrossRef
123. SancakY, PetersonTR, ShaulYD, et al.The Rag GTPases bind raptor and mediate amino acid signaling to mTORC1. Science2008;320:1496. CrossRef
124. SancakY, Bar‐PeledL, ZoncuR, et al.Ragulator‐Rag complex targets mTORC1 to the lysosomal surface and is necessary for its activation by amino acids. Cell2010;141:290. CrossRef
125. Bar‐PeledL, SchweitzerLD, ZoncuR, et al.Ragulator is a GEF for the rag GTPases that signal amino acid levels to mTORC1. Cell2012;150:1196. CrossRef
126. InokiK, LiY, XuT, et al.Rheb GTPase is a direct target of TSC2 GAP activity and regulates mTOR signaling. Genes Dev2003;17:1829. CrossRef
127. InokiK, LiY, ZhuT, et al.TSC2 is phosphorylated and inhibited by Akt and suppresses mTOR signalling. Nat Cell Biol2002;4:648. CrossRef
128. GaramiA, ZwartkruisFJ, NobukuniT, et al.Insulin activation of Rheb, a mediator of mTOR/S6K/4E‐BP signaling, is inhibited by TSC1 and 2. Mol Cell2003;11:1457. CrossRef
129. WangS, TsunZY, WolfsonRL, et al.Metabolism. Lysosomal amino acid transporter SLC38A9 signals arginine sufficiency to mTORC1. Science2015;347:188. CrossRef
130. RebsamenM, PochiniL, StasykT, et al.SLC38A9 is a component of the lysosomal amino acid sensing machinery that controls mTORC1. Nature2015;519:477. CrossRef
131. JewellJL, KimYC, RussellRC, et al.Metabolism. Differential regulation of mTORC1 by leucine and glutamine. Science2015;347:194. CrossRef
132. GwinnDM, ShackelfordDB, EganDF, et al.AMPK phosphorylation of raptor mediates a metabolic checkpoint. Mol Cell2008;30:214. CrossRef
133. LiY, InokiK, YeungR, et al.Regulation of TSC2 by 14‐3‐3 binding. J Biol Chem2002;277:44593. CrossRef
134. MaXM, BlenisJ. Molecular mechanisms of mTOR‐mediated translational control. Nat Rev Mol Cell Biol2009;10:307. CrossRef
135. TangH, HornsteinE, StolovichM, et al.Amino acid‐induced translation of TOP mRNAs is fully dependent on phosphatidylinositol 3‐kinase‐mediated signaling, is partially inhibited by rapamycin, and is independent of S6K1 and rpS6 phosphorylation. Mol Cell Biol2001;21:8671. CrossRef
136. WangX, SatoR, BrownMS, et al.SREBP‐1, a membrane‐bound transcription factor released by sterol‐regulated proteolysis. Cell1994;77:53. CrossRef
137. DuvelK, YeciesJL, MenonS, et al.Activation of a metabolic gene regulatory network downstream of mTOR complex 1. Mol Cell2010;39:171. CrossRef
138. JonesJR, BarrickC, KimKA, et al.Deletion of PPARgamma in adipose tissues of mice protects against high fat diet‐induced obesity and insulin resistance. Proc Natl Acad Sci U S A2005;102:6207. CrossRef
139. KimJE, ChenJ. regulation of peroxisome proliferator‐activated receptor‐gamma activity by mammalian target of rapamycin and amino acids in adipogenesis. Diabetes2004;53:2748. CrossRef
140. ZhangHH, HuangJ, DuvelK, et al.Insulin stimulates adipogenesis through the Akt‐TSC2‐mTORC1 pathway. PLoS ONE2009;4:e6189. CrossRef
141. WestergaardH, DietschyJM. The mechanism whereby bile acid micelles increase the rate of fatty acid and cholesterol uptake into the intestinal mucosal cell. J Clin Invest1976;58:99. CrossRef
142. MihaylovaMM, ShawRJ. The AMPK signaling pathway coordinates cell growth, autophagy and metabolism. Nat Cell Biol2011;13:1016. CrossRef
143. TowleHC. The metabolic sensor GCN2 branches out. Cell Metab2007;5:85. CrossRef
144. EfeyanA, ZoncuR, SabatiniDM. Amino acids and mTORC1: from lysosomes to disease. Trends Mol Med2012;18:524. CrossRef
145. LaplanteM, SabatiniDM. An emerging role of mTOR in lipid biosynthesis. Curr Biol2009;19:R1065. CrossRef